Another important milestone for the project has been published in the journal Nature Catalysis, in a paper that describes one of the promising pathways developed in FutureAgriculture to bypass the photorespiration.
The team led by Tobias Erb from the Max Planck Institute for Terrestrial Microbiology has developed a synthetic photorespiratory bypass that represents an alternative to natural photorespiration. Together with the Consortium, the Erb’s lab has designed the so-called tartronyl-CoA (TaCo) pathway that is much shorter than natural photorespiration and requires only 5 instead of 11 enzymes. The perhaps greatest benefit of the TaCo pathway is that it fixes CO2 instead of releasing it, as it happens in natural photorespiration. As a result, the TaCo pathway is more energy-efficient than any other proposed photorespiratory bypass to date.
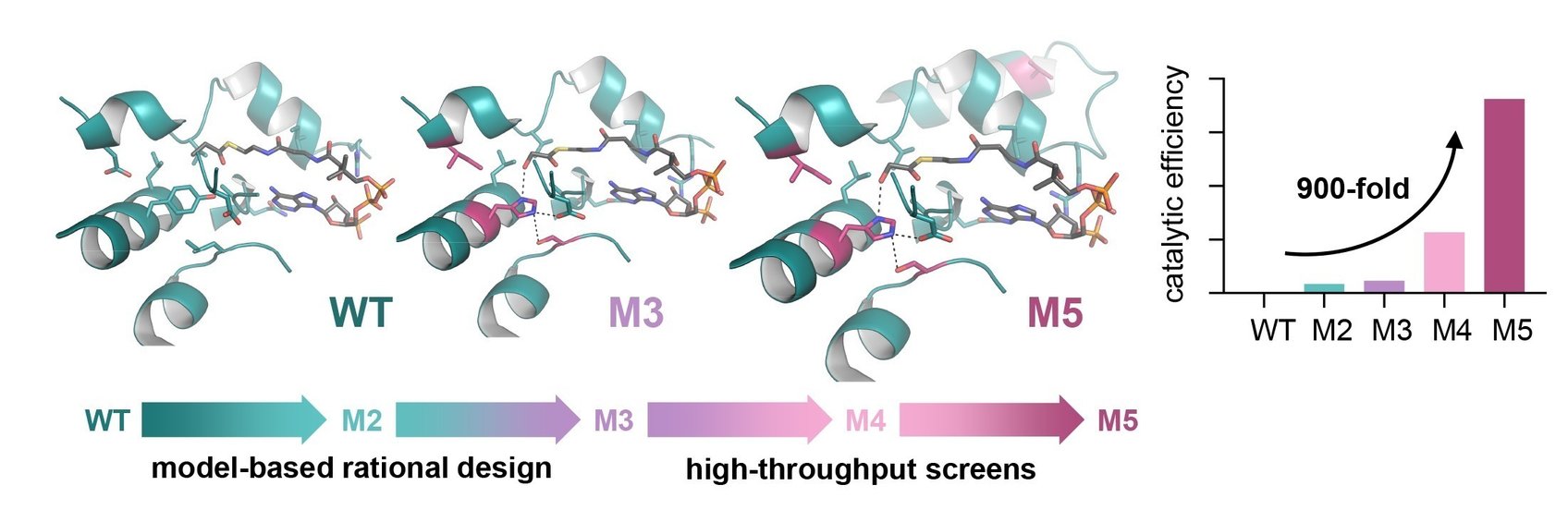
Building the TaCo pathway was a scientific journey that has led the researchers from the computational model through enzymatic engineering, microfluidic high-throughut screening, cryo-EM-technology towards the successful in vitro implementation of a new-to-nature metabolic connection that opens up new possibilities for CO2 fixation and the production of value-added compounds. “The main challenge in realizing the TaCo pathway was to find all the required enzymes,” Marieke Scheffen, Postdoctoral researcher in Tobias Erb`s group and lead author of the study, recalls. “It meant that we had to look for enzymes that perform similar reactions and then “teach” them to perform the desired reaction.”
For more information please check the original news by MPI and the scientific paper.
Reference: